Laser Particle Acceleration
Particle Acceleration
Short pulse, high-intensity lasers can be used to accelerate particles by creating large-amplitude electric field structures within gaseous or solid-density plasmas. The field strengths reached can be thousands to millions of times higher than possible with conventional RF technology, opening up the prospect of cheap, compact desktop accelerators for a wide range of industrial and medical applications. Laser-electron acceleration at the GeV level has been achieved by a number of labs worldwide and is now being seriously considered by CERN as a potential future collider technology.
Because of their higher inertia, ions are much harder to accelerate, and require a somewhat different approach, relying on laser-maintained charge separation as a way of supplying a 'DC' accelerating field. Our work on this concept has focussed on so-called 'mass-limited' targetry, such as nanoclusters or thin foils, in which the entire structure is pushed by radiation pressure to relativistic velocities (see figure). The advantage of this scheme compared to the more conventional 'sheath' acceleration from the rear side of micron-thick foils is that the proton spectrum is quasi-monoenergetic, or beam-like rather than exhibiting a broad thermal spread.
Tandem-pulse Laser Wakefield Acceleration
Since Laser Wakefield Acceleration (LWFA) was first introduced (T. Tajima and J. M. Dawson, Laser electron accelerator, Physical Review Letters 43(4), 267 (1979)), numerous electron acceleration schemes have been proposed aiming for higher electron energy while maintaining low emmitance and energy spread. For higher beam quality, control over electron injection is necessary, which can be achieved through different injection schemes, e.g. ionization injection, self-injection or injection via the density gradient (J. Faure, Plasma injection schemes for laser-plasma accelerators, CERN Yellow Rep. 1, 143 (2016)).
A recent study has shown that two co-propagating laser pulses of the same frequency separated by an appropriate delay and energy fraction could decrease the self-injection threshold and at the same time improve the beam quality (Z. M. Chitgar et al., Electron self-injection threshold for the tandem-pulse laser wakefield accelerator, Physics of Plasmas 27, 023106 (2020)).
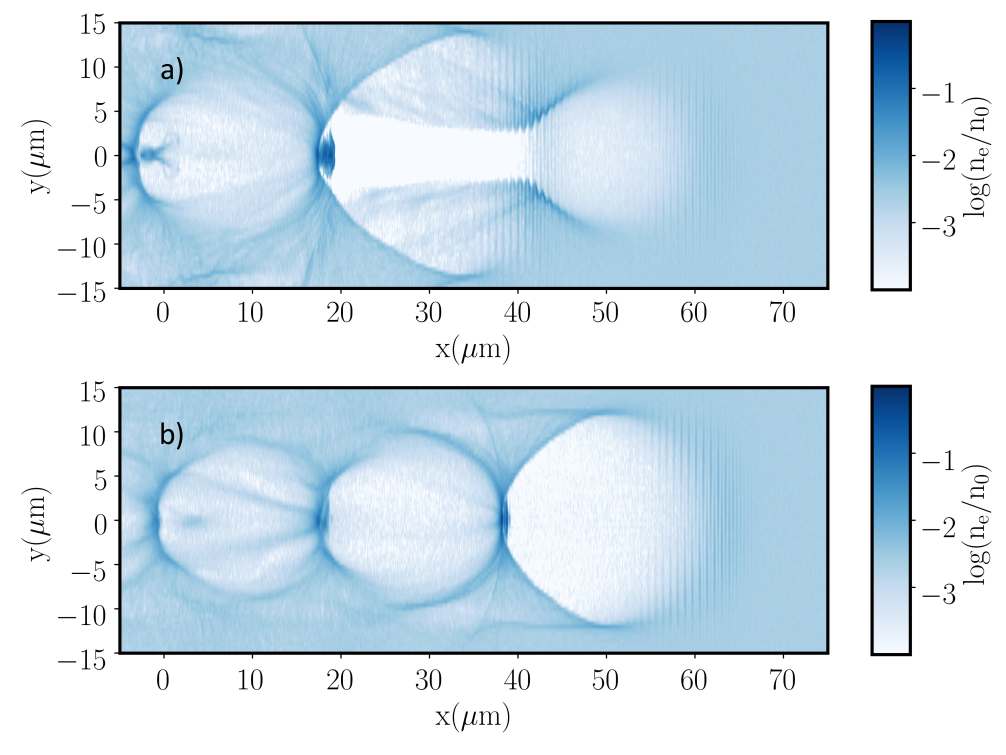
This scheme works similarly to the nonlinear blowout regime (A. Pukhov, and J. Meyer-ter-Vehn, Laser wake field acceleration: the highly non-linear broken-wave regime, Applied Physics B 74.4, 355-361 (2002)), where the electrons are pushed away from the high intensity region of the laser pulse forming a cavity before returning to their original positions. Depending on their momenta, some electrons may get injected into the cavity and then accelerated by the strong electric field created within the cavity (with a field gradient of the order of 100 GeV/m). This effect is strongest when pulse duration is matched to the electron plasma period τL∼ωp−1 and the laser intensity exceeds 8.5×1018 W/cm2, the so-called matching condition. Using a second laser pulse placed at the back of the first cavity reduces the laser intensity threshold for injection and results in the formation of a well-evacuated cavity with higher field gradient.
Using this scheme, an electron bunch of 0.29 nC charge is accelerated up to 536 MeV within a target length of ∼800 μm. The peak of the energy is at 395 MeV with spread of ΔE/E=12.7%, whereas the matched single-pulse scheme yields a beam with a charge of 0.22 nC and a maximum energy of 450 MeV with a larger FWHM bandwidth of ΔE/E=33% and a peak at 300 MeV. Adapting the new matching condition could potentially further optimize the bunch quality in the tandem-pulse scheme.
References
T. Tajima and J. M. Dawson, Laser electron accelerator, Physical Review Letters 43(4), 267 (1979)
J. Faure, Plasma injection schemes for laser-plasma accelerators, CERN Yellow Rep. 1, 143 (2016)
Z. M. Chitgar et al., Electron self-injection threshold for the tandem-pulse laser wakefield accelerator, Physics of Plasmas 27, 023106 (2020)
A. Pukhov, and J. Meyer-ter-Vehn, Laser wake field acceleration: the highly non-linear broken-wave regime, Applied Physics B 74.4, 355-361 (2002)